Silica in Poaceae
Contents
Silicon for algae
Tilapia naturally thrive on diatoms and Spirulina, which are algae. Diatoms are the world’s largest contributors to biosilicification.[8] Most diatom species have an obligate requirement for silicon for cell wall formation.[9] Particularly diatoms that thrive in saline, alkaline conditions generally require high silicon:phosphorus ratios, including Anomoeoneis sphaerophora, Rhopalodia species, Melosira granulata [10], Nitzschia species [11] and Synedra ulna [12]. Compost may be used for supplying nutrients for the cultivation of Spirulina and diatoms. Compost may be derived from composting toilets. Such composting toilets frequently need the addition of dried plant material (sawdust, dried grasses or -leaves) as a carbon source. Silicon is the second most abundant element in soil. To add silicon along with the carbon, the used plant material should be silicon accumulating.
Silica in plants
Plants may take up silicon from the soil if the pH is below 9. Biogenic silica is known to alleviate plant stressors such as high temperatures and drought.[13] There may be no relationship between soil type and plant Si concentrations, but with temperature and precipitation.[14] Water use per biomass is lowest in regions with a high rainfall to evaporation ratio. Silicification in non-grass species is probably less dependent on soil silica availability [15] and water availability.[16].
Grasses
Leaf silica concentration in tall grass species increases with watering [17] and with age.[18] Blade silica content is higher after the grass has been defoliated.[19] Silica levels are lowest in the stem fraction. The level of silica in grasses may co-depend on the level of silica in the soil, availability of water [20], the pH of the soil and temperature. Grasses adapted to hot climates and atmospheres low in carbon dioxide (C4 grasses), usually have lower silica contents than C3 grasses (cool-season grasses). There is no consistent relationship between silica content and annual precipitation.[21] Grasses may also solubilize silicate from clay. [22] In perennial grasses, the main source of silica is from monosilicic acid (soluble silica) in the water. Silica levels in grasses are highly influenced by the monosilicic acid contents of the soil. As a result, clay soils result in higher silica contents in grasses than sand.[23]
Wheat (Triticum spp.) are grass species and may contain 0.3 to 10% silicon.[24] Triticum aestivum may contain 2.5%, Triticum boeoticum 2.5%, Triticum dicoccoides 1.2% and Triticum percicumx 1.6% silica. Rice (Oryza sativa) may contain 4.2% silica. [25] Pennisetum purpureum (Elephant grass, a Panacea) may contain 0.85% silica [26] Particularly 'Millet-Paniceae' may be effective silica accumulators, such as some Brachiaria and Panicum species.
Brachiaria
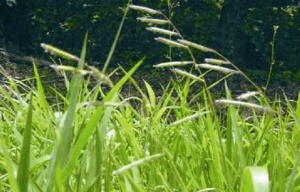
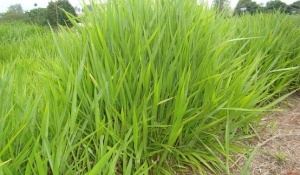
Brachiaria species ('Signal grass') are C4 ('Millet') grasses that grow fast.[27] Brachiaria brizantha may contain 1.38% silicon [28] Brachiaria brizantha adapts poorly to poorly drained soils.[29]
Particularly Brachiaria decumbens (aka Urochloa decumbens, aka Surinam grass / Signal grass) may be considered silicon accumulating (3.6% in adult leaves[30]) and resistant to droughts.[31] It occurs in Burundi, Rwanda, Democratic Republic of Congo, Kenia, Tanzania and Uganda.[32] Silica levels in Brachiaria decumbens are highest in mature leaves, particularly on the upper epidermis of the grass leaf blades.[33] Yields of 10 tonnes (rainfed) and 19.5 tonnes (irrigated) dry matter/ha/year have been reported.[34] This may in part be due to its capacity of evapotranspirative adaptation in relation to the dynamic between water-transpirating and water absorbing surfaces as well as surface conductance sensitivity to soil transfer and yielding capacity.[35] In phosphorus-deficient conditions, Brachiaria decumbens very effectively increases phytase and phosphatase activity to utilize inositol hexaphosphate in soil.[36] Brachiaria decumbens may grow in low fertility, low pH (as low as pH 3.5) soil, while it does not respond to lime, but responds strongly to N and P fertiliser. It cannot persist on heavy clays subject to waterlogging, as its root system is very fine with low tolerance of poor drainage. Brachiaria decumbens stays green well into the dry season. It is most productive on open grasslands in the lowland humid tropics with rainfall >1,500 mm annually and a dry season of up to 5 months. It can tolerate short-term flooding and prefers temperatures above 19ºC. It is very tolerant of heavy grazing but less tolerant under reduced light than in full sun.[37] Grasses decompose relatively slowly. The rate of decomposition of organic matter from Brachiaria decumbens is considered intermediate. Remaining polyphenols may serve as an indicator of litter decomposition.[38] Chopping reduced contents of acetic acid, propionic acid and butyric acid and increases NH3-N content.[39]
Brachiaria decumbens spreads by seed dispersal. It is an apomictic tetraploid that reproduces mainly by facultative apospory.[40] Reproduction approximates a diploid-tetraploid-(di)haploid reproductive cycle that does not involve triploids. Natural Brachiaria decumbens tetraploid populations are widespread, whereas diploid populations are rare. Tetraploids are considered obligate aposporous apomicts, propagating clonally, with the absence of genetic variation.[41] Even though Brachiaria decumbens is considered obligate apomitic, completely sexual accession does occur. The sexual accessions are diploids (2n = 2x = IS).[42] Brachiaria decumbens may use allelopathy to outcompete other grasses. Germinating seeds and the aqueous leachates of Brachiaria decumbens reduce the germination of other grass species, such as Phalaris canariensis, Lactuca sativa and Melinis minutiflora.[43] Brachiaria decumbens has abnormal nucleolar cycle behavior.[44]
Brachiaria decumbens contains several saponins and sapogenins [45], including 3-spirostanols [46], mainly diosgenin, and smilagenin, sarsasapogenin [47], epismilagenin and episarsasapogenin.[48] Sapogenins are saponins that may contain a steroid frameworks as their key organic feature.[49] In tilapia, saponins may increase growth and LH release [50], inhibit intestinal magnesium uptake [51] and diminish egg production by females.[52] In sheep, the saponins are metabolized in the rumen, and absorbed sapogenins are excreted in the bile as calcium salts of the sapogenin glucuronides.[53] Saponins may be degraded by aerobic microorganisms.[54] [55] Composting involves degradation by aerobic microorganisms. So that decomposted Brachiaria decumbens leaves may be a safe source of silica for diatoms in water that also ends up in tilapia ponds.
Panicum

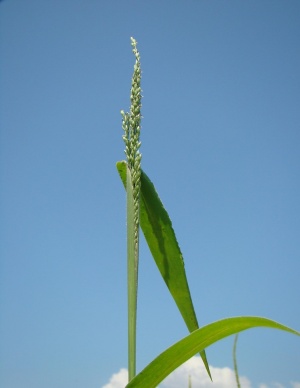
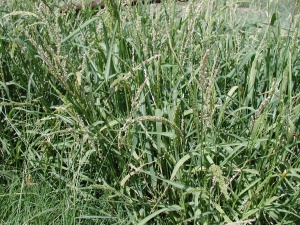
Panicum species ('Panic grass') are large tropical ('millet') grasses. Panicum maximum ('Buffalo grass') may contain 1.07% [56] or 0.5% silica.[57] Panicum virgatum ('Switch grass') may contain 1.5% [58] or 2.0% silica. Panicum commutatum may contain 4.2% and Panicum texanum may contain 8.0% silica [59] or 7%.[60]
Panicum texanum (aka Brachiaria texana or Urochloa texana, aka Buffalo grass, Colorado grass, Texas signal grass or Texas millet) has up 2 cm wide leaves (7.5-28 cm long) that are covered with soft minute hairs on both surfaces. Its stems may be up to 136 cm long, and the grass may grow 80 cm high.[61] It is a common troublesome weed in many commercial crops, including field corn (maize)[62] and peanut.[63] It grows best at higher temperatures. It may grow well at 30°C during the day and 24°C during the night. Its low temperature threshold for growth is ~11°C. Panicum texanum attains 25% of its maximum growth at an average daily temperature of 21°C, 50% at 24°C and 75% at 26°C. Partitioning of biomass into stems increases with higher nocturnal temperatures.[64] Panicum texanum may produce 53,000 seeds per plant.[65] (and therefore used by bird-enthusiasts[66]) It grows along streams, in open fields, on prairies and on deep moist soils, southern USA and Mexico. Both annual and perennial. It is used for hay.[67] It is best adapted to well-drained sandy soils, and usually produces 23,000 seeds per plant.[68] Vegetative stage Panicum texanum may contain 16% crude protein. It may contain 11% crude protein at flower/boot stage and 8% crude protein at fruit/head stage. Subsequent invitro dry matter digestibility may range from 74 to 52%.[69] Panicum texanum occurs in Queensland and New South Wales (Australia), Arizona, California. New Mexico, Texas. Alabama, Arkansas, Florida, Georgia, Louisiana and South Carolina (USA) and North-east Zacatecas (Mexico).[70] Panicum texanum is preferentially consumed by quails.[71]
Other Poaceae
Wetland Gramineae may accumulate up to 15% dry weight silica.[72] Besides grasses, Pleioblastus chino 5.1% (a bamboo species) may contain 5.1% silica, Marchantia polymorpha (common liverwort or umbrella liverwort) may contain 5.5%, Eleocharis uniglumis (spikesedges in aquatic or mesic habitats) 6.7% and Arundinaria gigantea (also a bamboo species) may contain 8.8% silica. [73]
Arundinaria gigantea
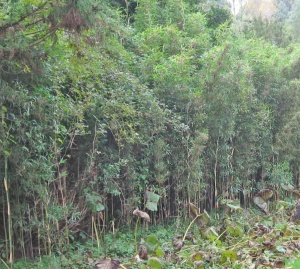

Arundinaria gigantea leaves may contain 8.8% [74] or even up to 18.76% silica[75] It is not a wetland specie [76] but thrives in sandy, well-drained mineral soils.[77] Arundinaria gigantea (aka Giant cane, River cane or Canebrake bamboo). Arundinaria gigantea var. tecta or Arundinaria tecta (Switch cane) is smaller (up to 2 m high) and adapted to more aquatic conditions. [78] Colonies of Arundinaria tecta flower every four or five years, those of Arundinaria gigantea every 40- 50 years.[79]
Arundinaria gigantea is a perennial evergreen Bamboo from hard, tough rhizomes, growing up to 9 m high. Its flowers have both male and female organs and are pollinated by Wind. It grows well in sandy, loamy and clay, well-drained, moist soils. It can grow in acid, neutral and basic soils, and is indifferent of (the lack of) direct sunlight exposure. Arundaria gigantea is intolerant of drought, requiring abundant moisture and plenty of organic matter in the soil. It prefers warm, humid, damp conditions.[80] It has low calciumcarbonate tolerance.[81] Arundinaria gigantea may monopolize newly freed space and resources through rapid clonal growth.[82] Intense rains cause transportation of sediments. Giant cane very effectively filters sediment because its dense population slows the velocity of surface runoff and because its fine root system increases soil porosity (promoting infiltration) and tightly binding soil particles.[83] Giant cane may reduce ground water nitrate levels by 90%, through plant assimilation, denitrification and by upwelling ground water through the porous soil.[84]
In bamboo stands, culms are connected to each other through rhizomes and regenerate by shooting sprouts. New sprouts attain full size within a few months.[85] Propagation of giant cane can be carried out either sexually by seeds or asexually through transplanting culms. Because clusters of flowering culms are often monoclonal, sexual reproduction is often characterized by gregarious flowering, followed by death of the flowering culms and possibly of the attached rhizomes.[86] Hybridization occurs naturally among this species, with putative hybrids.[87] Seeds are often low in viability (82–95% viable[88]), and rarely available because Giant cane rarely flowers. The seeds need to be surface sown. Compost needs to be kept moist. Germination is usually swift, but may also take up to 6 months. Maximizing seed germination offers the ability to exploit seed produced on existing canebrakes. Of the six temperature regimes tested on two populations, maximum germination occurred using roll towels, under a 35/25 or 30/20°C temperature regime.[89] The seedlings need to grow in light shade, in a high fertility sandy medium. [90] Levels of 5 g./L nitrogen significantly increases seedling growth when plants are grown under non-shaded conditions. Giant cane growth is enhanced with increased light levels.[91] Fertilizing (N, P, and K) establishing giant cane may reduce mortality, and periodic burning can increase density and spread of this species, which should promote establishment over the long-term.[92]
Propagation by digging and transplanting culms and allowing for subsequent spreading of rhizomes is labor intensive and cumbersome. Using rhizome cuttings to produce planting stock requires a method for the successful culm production. Surface-planted rhizomes produced 75 culms compared to the 26 culms produced by buried rhizomes. Surface-planted rhizomes with 10+ internodes averaged the fewest number of internodes (7.9) needed to produce at least 1 culm. In other words, cutting rhizomes up into sections with fewer internodes (as was the case in the 2 and 4 internode pieces) resulted in fewer culms produced for a given amount of available rhizome tissue. Exposure of rhizomes to sunlight during propagation increases the number of culms that are produced, particularly for those that are greater than 10 internodes long. Rhizomes become photosynthetic and thus provide energy needed to help stimulate production and the growth of culms. Exposure to light of normally shaded or light-excluded tissues may also stimulate bud break from dormant buds, as is seen in the production of epicormic shoots in trees. Relatively small rhizome sections (22 to 30 cm) offer the advantage of being able to set out more propagules with the same amount of collected plant material. Additionally, smaller rhizomes are easier to handle for outplanting.[93] Macropropagation from rhizome sections derived from stock maintained in pot-in-pot container production allows for relatively easy harvest. Rhizome sections 2-3 nodes in length soaked for 60 minutes in warm water or 1000 ppm BAP (6-benzylaminopurine) showed greatest shooting yield. Individual mother plants have the potential to yield 400 viable clones.[94]
It is recommended that rhizome propagules be yellowish, 38 to 102 cm long, with at least 10 good buds. Rhizomes from younger planted stands have higher survival in the greenhouse; having a higher number of buds may lead to a higher probability of a bud to produce a culm. Potential culm size (height and diameter) is a reflection of available food reserves in the rhizomes. Using longer rhizomes provides more available non-structural carbohydrates. With the development of new shoots, carbohydrate concentration in the rhizoma decreases. Planting rhizomes directly in the field requires less effort, time, and resources than initial growing in the greenhouse. Both origin (putative genotype) and stand development (collection source) influence survival and growth of culms generated from rhizomes. Obtaining stock directly from natural stands tends to produce taller culms but survival was similar to rhizomes collected from plantings. While natural stand rhizomes are larger in diameter and provide taller initial culms, they do not provide the same number of buds per unit length as a plantation source. Having buds that form both new culms and new rhizomes is desirable and choosing propagules with fewer buds lowers any chances of that happening. Macropropagation success in containers can increase with rhizomes that have more buds per unit length and greater diameter.[95]
Low-silica grasses for Armyworms
Armyworm eggs may be used to mass-rear green lacewings, and its pupa and young larvae to feed tilapia. High silica in grasses and sedges inhibits Armyworm growth. The addition of silicon to the soil increases the size, shape, number and density of spines and phytoliths in the leaves of grasses.[96] And when defoliated by herbivores, silicate contents may increase 4-fold in response [97], but not nearly so much in grasses clipped with scissors.[98][99] Particularly in Africa the effects of grazing on silica contents are great, but not the effects of burning by fire.[100] Silica is deposited as phytoliths in leaves. These are harder than tooth enamel.[101] Phytoliths may also disrupt microbial action in the gut.[102] Spodoptera exempta cannot easily adapt to physical defences such as silica. Silica in the leaves of grasses (2-5% of dry leaf mass [103]) acts as a defence. Even with short-term exposure, but also progressively impacting with time, silica reduces the conversion efficiency of food into bodymass, and the amount of nitrogen absorbed from their food, leading to reduced growth rates. Exposure to silica-rich diets also caused an extremely rapid increased mandible wear.[104] This results in mechanical protection of resources in chlorenchyma cells. As a result of this (and of the disrupting influence of phytoliths on microbial action in the gut), less chlorophyll is released after grinding and more chlorophyll is retained after passing through the gut.[105]
Low silica grasses/sedges are Agrostis scabra (Ticklegrass, a tumbleweed ; 0.3% silica), Anthoxanthum odoratum (0.6% ; Vanilla grass in Eurasia), Arrhenatherum elatius (0.9% ; tall oatgrass in temperate Europe), Carex aquatilis (arctic and temperate water sedge), Carex flavicans, Carex subspathacea, Chasmanthium sessiliflorum (0.7% ; longleaf woodoats in temperate USA), Cymbopogon citratus (0.8% ; lemon grass in South-east Asia), Cyperus alopecuroides (aquatic tropical/temperate sedge), Dactylis glomerata {0.6% ; a cool-season perennial C3 bunchgrass), Juncus roemerianus (0.2% ; black needlerush in salt marshes and estuaries), Puccinellia phryganodes (arctic and temperate saltgrass), Scirpus tabernaemontani (0.1% ; temperate grassweed), Spartina alterniflora (0.7% ; Smooth Cordgrass in estuarine salt marshes), Spartina patens (0.8% ; saltmeadow cordgrass in brackish coastal marshes), Sporobolus cryptandrus (0.7% ; Sand dropseed in prairie), Stipa comata (0.7% ; needle/feather grass in savanna/prairie), Triticosecale spp.(0.1% ; Triticale, a wheat hybrid), Zea mays (0.8% ; maize), Zoysia japonica (0.3% ; creeping grass in temperate regions) and Setaria sphacelata (South African pigeon grass aka African bristlegrass aka Golden millet).[106]
Grasses that utilize water more efficiently, have lower silica contents. As a result, perennial C4 grasses such as Prairie cordgrass (Spartina pectinata), Switchgrass (2.0% ; Panicum virgatum), Big bluestem (1.4% ; Andropogon gerardii) and Prairie sandreed (Calamovilfa longifolia) contain relatively little silica. [107]Panicum coloratum (White buffalograss; a medium-high grass) may contain 1.21% silica.[108] It is drought-tolerant and does well in hot climates. Other perennial C4 grasses are Yellow Indiangrass (Sorghastrum nutans, 2.0% silica) and Bermudagrass.
Cynodon dactylon



Bermuda grass (Cynodon dactylon) is also a perennial C4 grass. Grasses preferred by Armyworm (Spodoptera exempta) larvae are sweet grasses in the genus Cynodon. [109] Silica contents in Bermudagrass may be 0.15% [110] or 1.5%.[111] Silica contents may be lower when the grass grows in sand, and higher when growing in clay.[112] Bermuda grass is rare in Ghana, but abundant when present (up to 60 cm tall), does not tolerate shadow and prefers sandy, muddy and well drained soils. It is frequent in Mali, Ivory Coast and Nigeria. [113]. When supplemented, silica accumulation in Bermudagrass is high, but post-supplemental retention is low.[114] The higher the nitrogen content of the leaves, the higher the Armyworm fecundity. In general, Armyworm fitness is hardly affected by leave nitrogen levels.[115] Net reproduction rate of Spodoptera exempta is greater on Bermuda grass (Cynodon dactylon) and maize than on Kikuyu grass, Pennisetum clandestinum, Guinea grass, Panicum maximum and Setaria plicatilis.[116][117] Larvae offered a choice of maize and Bermudagrass showed a preference for Bermudagrass. Survival was higher on Bermudagrass than on maize but the developmental time was longer.[118]
Bermuda grass may spread quickly via creeping stems (i.e. stolons and rhizomes).[119] Rhizomes (root-like stems) can penetrate 40-50 cm in clay soil and 70-80 cm in sand. It is foliage dense and 10-40 cm tall (rarely to 90 cm). Bermuda grass does best in relatively fertile, well-drained soils with pH over 5.5 with mean daily temperatures above 24°C (Optimum temperature for growth is 35°C[[120]]). It occurs over an average annual rainfall range of 625-1,750 mm, up to 4,300 mm. It is not shade tolerant. It is very drought tolerant by virtue of rhizome survival through drought-induced dormancy over periods of up to 7 months. Tolerates at least several weeks of deep flooding.[121]
Bermuda grass may be cut when 30-40 cm tall or every 4-6 weeks, usually when in full bloom. 4 cuttings per year are possible. A stubble height of 5-10 cm gives good regrowth and maintains sward density. Renovate by ploughing or discing when sod-bound. [122] Harvesting Bermuda grass at 4 weeks (close cut) increases crude protein in comparison with a 8-week cut. Crude protein varies from 8.3% in mature to 14% in young grass, up to 22% in nitrogen-fertilized grass. For protein production, nitrogen utilization from fertilizer (eg manure) begins to decline with 450 kg/ha. Dry matter may contain 1.1% oxalic acid. When sod-bound, renovate by ploughing or discing.[123]
Bermuda grass may be propagated by seed or vegetatively (turfs or stolon/rhizome pieces). Normally sown at 5-10 kg/ha dehulled seed, the higher rate being used for more rapid cover. Seed is best sown onto a very well prepared, fine, weed-free seedbed and rolled in. Seedlings usually root down quickly. Improved varieties are usually planted vegetatively due to low seed set or to avoid genetic drift. Turfs or sprigs can be planted at 3.5-7 m³/ha (40-80 bu/ac) or on a 90 cm (or less) grid, into a roughly or well-prepared seedbed, but rolling is still essential. Bermuda grass responds well to improved fertility, with applications of a minimum of 10 kg/ha/month N and up to 60 kg/ha/month N necessary for moderate to high productivity.[124]